The fifth generation (5G) technology standard has been in a continuous evolution for years now. With the standardization of 3GPP Release 16 and the ITU deeming the 3GPP 5G New Radio (NR) standard to officially meet the IMT-2020 standards originally set in 2015, it seems the technological leaps towards 5G can be much more readily realized.
There are myriad of advanced protocols, radio technologies, and infrastructural enhancements that are leveraged towards achieving the key performance indicators (KPIs) set by the IMT-2020 standard. This article focuses on 5G backhaul and how latency and throughput requirements can be achieved through specific gNB types and backhaul technologies.
What's the point of 5G?
In its most basic form, the goal of 5G is to provide the appearance of seamless connectivity for the user despite the apparent obstacles. These obstacles vary depending upon the scenario, in an urban environment this can mean congestion and interference within the spectrum being utilized. In a rural environment, this can mean a generalized lack of access due to the inability to reach the local base station. It can also mean a slow connection from streaming data-hungry applications such as HD video, augmented/virtual reality (AR/VR), autonomous driving, or tactile internet (TI) usage. Another use case could also include an industrial application attempting to receive data from thousands of sensor nodes in order to track/monitor machine equipment all without a hitch.
All of these above scenarios have with it, its respective design considerations in base station architecture, backhaul, and spectrum usage. For this reason, many of the design parameters and standards released revolve around the 5G key performance parameters. The KPIs form the nominal and upper limits for the different 5G use cases. The use cases are typically defined as follows:
- • Enhanced Mobile Broadband (eMBB)
- • Ultra-Reliable Low Latency Communications (uRLLC)
- • Massive Machine Type Communications (mMTC)
Of all the various types of streaming (e.g., audio, web browsing, social media, software downloads, etc.), video streaming is expected to account for 74 percent of mobile data traffic by 2024[1]. Moreover, it predicted that by 2023, 70 percent of the global population will have mobile connectivity, bringing the number of networked devices to nearly 30 billion ― over 60 percent more than there were in 2018[2]. For this reason, the eMBB use case scenario revolves around meeting high data-rate demands despite high capacity.
On the other hand, mMTC the simultaneous connection of tens of billions of devices that typically, are not transmitting/receiving large amounts of data and therefore involve relatively low throughputs. This is due to the hike in connected internet-of-things (IoT) devices ― an expected 50 billion devices are expected to be IoT-enabled by 2030[3]. As made apparent by its name, uRLLC scenarios require low-latency communications reliably. This is applicable, for instance, in medical/wearable, mission critical (military), public safety, or factory automation applications where any delay or down-time in communications yields the risks of factory down-time, damaged equipment, or loss of life.
Meeting the key performance indicators
The IMT-2020 (International Mobile Telecommunications-2020) standardizes the requirements of a 5G network and was issued by the ITU (International Telecommunication Union) in 2015. However, this is a collaborative effort between the ITU and the other major cellular standards organization of 3GPP (3rd generation partnership project). As recently as July 2020, the ITU has concluded that the 3GPP 5G (Releases 15 and 16) fulfill both the 5G Phase 1 and Phase 2 requirements, making it viable to meet the technological requirements of the IMT-2020 standard[4]. The general KPIs of 5G as set by IMT-2020 can be seen in Figure 1.
Figure 1: 5G requirements.
There are a number of technological improvements and milestones that have been developed in order to meet the 5G KPIs. Figure 2 lists some of the 3GPP enhancements and how the various radio aspects and system aspects help meet the needs of all industry verticals and the three major use cases.
Figure 2: Vertical industry integration with 3GPP Rel-16 [4]. [Image Credit: ITU]
Key enabling technologies for 5G
There are some major technological “pillars” that allow for the 5G KPIs in various use cases, including massive MIMO and the use of the millimeter-wave spectrum. Moreover, the augmentation of the current 4G infrastructure with 5G new radio (NR) in Non-standalone (NSA) 5G is a critical phase in ultimately meeting true 5G. It is beyond the scope of this article to dive into detail about these technologies and their implementation in the 5G infrastructure. However, the connection of the various 5G gNB’s to the 5GC is one key challenge in meeting the throughput, latency, and spectral efficiency requirements. This is where the 5G backhaul architecture plays a key role. The type of backhaul structure varies depending upon a number of parameters. Figure 3 shows some basic ranges used by the 5G Infrastructure Public Private Partnership (5G-PPP) to qualify basic KPIs in order to classify their six major use cases (i.e., dense urban, broadband, connected vehicles, smart offices, IoT, TI)[5].
Figure 3: Parameters to consider around various 5G use cases[5].
Nuts and bolts of 5G backhaul: the functional split
The 3GPP next generation radio access network (NG-RAN) architecture is a disaggregated network topology where a 5G NR base station (gNB) has its functionality split between a centralized unit (CU) and one or more distributed units (DUs) with the additional possibility of a separate radio unit (RU).
Typically, the CU processes non-real time protocols while the DUs are meant to process PHY level protocols and real-time services to meet the low latency needs of specific applications. Deployment scenarios vary depending upon the type of base station and mobile network operator (MNO). In each of these cases, the fronthaul, midhaul, and backhaul network will vary. Table 1 lists the various topologies of the RAN deployment scenarios. In each of these, the use of a fronthaul or midhaul network varies depending upon the type deployment. For instance, a hotspot or a small cell could have an integrated RU, DU and CU where there would be no fronthaul, or midhaul, but only backhaul to the 5GC.
Table 1: RAN deployment scenarios
As shown in Figure 4, the functional split within the gNB can either be a low layer split (LLS) or a high layer split (HLS) within the open systems interconnection (OSI) model ― a model showing the functional layers within a networking system. The HLS generally allows for lower latency and higher throughput communications over a LLS. The intra-PHY split, or a functional split within the physical layer, has the benefit of supporting NR features as carrier aggregation (CA), network multiple input multiple output (MIMO), and coordinated multipoint (CoMP), and dual-connectivity (DC). Traditionally, the CPRI protocol was used to support communication between the baseband unit (BBU) and the remote radio head (RRH). However, since CPRI does not scale with gNBs equipped with an intra-PHY split, the enhanced CPRI (eCPRI) protocol has since been standardized to support the functional decomposition for fronthaul (communication between the RU and DU).
Figure 4: Split points between CU and DU functions with a (a) high layer split (b) low layer split, and (c) cascaded split[6]. [Image Credit: ITU]
These functional splits in the physical layer require coordination from the higher layers, making latency requirements very strict. For instance, 3GPP set limits on the Time Alignment Error (TAE), or the largest timing difference between any two NR signals for a specific configuration/transmission mode, on the order of a hundred nanoseconds for these NR signals as the frames of these signals in the radiated domain are not perfectly aligned in time[7]. This requires a high degree of synchronization as the maximum one-way frame delay for communications can go as low as 25 µs (uRLLC) with an end-to-end time synchronization of 1.5 µs[8].
Table 2 lists the one-way latency of various types of backhaul based on operator inputs listed in 3GPP standards as well as wireless backhaul in the sub-GHz and mmW frequencies[9]. The Hybrid ARQ (HARQ) process located in the MAC is enabled in 5G in order to meet the low latency requirements. This process requires a round trip time of 5 ms, corresponding to a 40 km fiber distance between the CU and DU[10]. In other words, there are a wide variety of NR signals and usage scenarios that involve both synchronized and unsynchronized signals between small-cells as well as between small cells and macro-cells. The xhaul should vary accordingly, where small-cells and macro-cells utilizing operations such as interference coordination, CA, and CoMP, can be enhanced through synchronized deployments of small-cell clusters and coordinated communications. This requires a specific gNB arrangement between the CU, DU, and RU, with a particular functional decomposition as well as an adequate backbone for backhaul (fiber or wireless).
Table 2: Backhaul technology and respective one-way latency and throughput[9].
The choice of backhaul technology: fiber or wireless
Just as the 5G utilizes a myriad of radio technologies and spectrum space to achieve seamless connectivity, the infrastructure for fronthaul, midhaul, and backhaul is flexible as well between wired and wireless technologies. Depending upon the area and usage scenario, there are known site configurations and types of gNBs that are utilized. As shown in Table 3, this can vary between a small-cell or macro-cell where the gNB could leverage the mmW spectrum, 5G MIMO, or even be an eNB[11][12]. Dense urban environments can achieve high capacities reliably through a heavy small-cell infrastructure with fiber access to the 5GC over short transmission distances for low-latency communications. Suburban and rural environments have the major issues of accessibility, in these cases high data rates can be achieved through wireless backhaul through a macro-cell and, more recently, through satellite backhaul with a Geosynchronous Orbiting (GEO) high throughput satellite (HTS) or low earth orbiting (LEO) constellations.
Table 3: Various coverage areas and their respective parameters[11][12].
In any case, 5G calls for an increased amount of deep fiber installations with the options of microwave and millimeter-wave backhaul. According to ABI research, fiber backhauling is set to grow to 39.6% of backhaul links for macro-cells by 2025 while microwave backhaul takes up 45.1% of that market share. The third largest predicted backhaul technology is millimeter-wave backhaul at 6.1%. Fiber deployments are often cost- and time-prohibitive for the initial phase of 5G. For this reason, Integrated Access Backhaul (IAB) was specified as part of the 3GPP Release 16 standard. This type of backhaul utilizes the already established gNBs (e.g., small cells, fixed wireless terminal, macro-cell, etc) to backhaul a signal through a series of hops, using the same or different frequency bands.
Meeting latency, throughput, and distance requirements in 5G with backhaul
The careful use of fiber and wireless xhaul is essential for meeting 5G throughput and latency requirements over vast distances and/or signal obstacles. This complex problem is solved through a myriad of techniques and technologies, including IAB, microwave backhaul, millimeter-wave backhaul, and the choice of fronthaul or midhaul (or both) for a LLS or HLS in a gNB.
Generally speaking, dense urban environments heavily utilize fiber small cells for backhaul and connectivity while more rural environments will rely on wireless connections via a millimeter-wave or microwave wireless link.
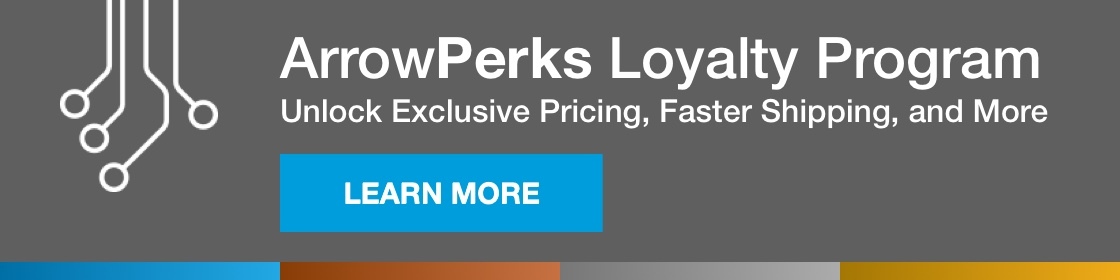
References
- https://www.ericsson.com/491e34/assets/local/mobility-report/documents/2018/ericsson-mobility-report-november-2018.pdf
- Cisco. “Annual Internet Report (2018–2023) White Paper.” Mar. 2020.
- https://www.statista.com/statistics/802690/worldwide-connected-devices-by-access-technology/
- https://www.itu.int/en/ITU-T/Workshops-and-Seminars/201807/Documents/3_Erik_Guttman.pdf
- 5G PPP, “Version 1: 5G PPP use cases and performance evaluation models.”
- ITU-T GSTR-TN5G, “Transport network support IMT-2020/5G.” Feb. 2018.
- 3GPP, “TS 38.104 V16.5.0:Technical Specification Group Radio Access Network; NR; Base Station (BS) radio transmission and reception.” 3GPP, Sept. 2020.
- ITU-T G.8271, “Network Limits for Time Synchronization in Packet Networks,” International Telecommunications Union, October 2017.
- 3GPP, “TR 36.932 V16.0.0; Scenarios and requirements for small cell enhancements for E-UTRA and E-UTRAN”, Tech. Rep., 3GPP, Jul. 2020.
- L. M. P. Larsen, A. Checko and H. L. Christiansen, "A Survey of the Functional Splits Proposed for 5G Mobile Crosshaul Networks," in IEEE Communications Surveys & Tutorials, vol. 21, no. 1, pp. 146-172, Firstquarter 2019, doi: 10.1109/COMST.2018.2868805.
- 3GPP, “TR 38.913 V16.0.0: Study on Scenarios and Requirements for Next Generation Access Technologies.” 3GPP, Jul. 2020.
- https://www.itu.int/en/ITU-R/study-groups/workshops/fsimt2020/Documents/2-Wireless%20X-Haul%20Requirements.pdf